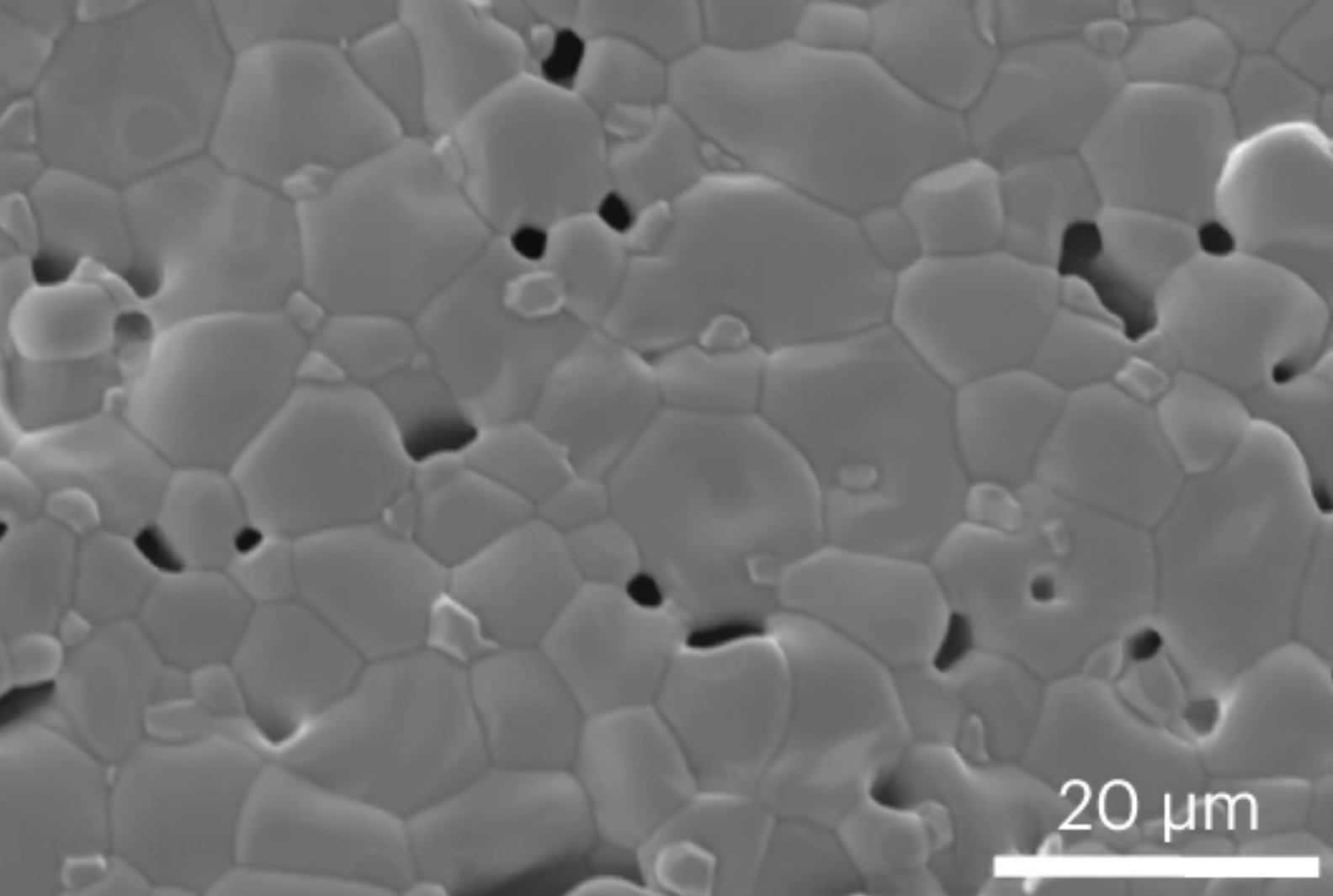
Additive manufacturing optimizes multifunctional chemically reactive systems
Tuning the properties of membrane and catalyst layers could offer a promising way to optimize reactors for fuel cells, electrolyzers and partial oxidation reactions.
Tuning the properties of membrane and catalyst layers could offer a promising way to optimize reactors for fuel cells, electrolyzers and partial oxidation reactions.
Written by Derek Smith
Ethylene is a chemical widely used as a feedstock for producing polymers and industrial chemicals. Oxidative coupling of methane (OCM) is a promising, energy-efficient way of creating ethylene from natural gas by reacting methane with oxygen. However, the method comes with its downsides. Too much oxygen can reduce product yields by converting ethylene into carbon dioxide. To circumvent this problem, some engineers are looking toward reactor designs that slowly introduce oxygen into the methane stream, which limits the exposure of the desired chemical products to oxygen. Such solutions, however, are more complicated and expensive than a conventional reactor, in which methane and oxygen would be simply fed together as gas-phase reactants over a catalyst material.
“For a more sustainable future, we need to push the envelope in the design of chemical conversion processes. Introducing tools of additive manufacturing can be helpful in realizing more complex designs” said Suljo Linic, the Martin Lewis Perl Collegiate Professor of Chemical Engineering and corresponding author of the study published in Nature Materials.
U-M researchers have designed a multifunctional membrane-catalyst reactor that minimizes reactions between oxygen and ethylene while maintaining high methane oxidation rates. The new design could make this energy-efficient method of creating products from natural gas more economical by overcoming the yield issues of previous OCM membrane reactor designs.
The team’s reactor uses a hollow fiber membrane-catalyst system made with multiple layers of gadolinium-doped barium cerate catalyst. The barium cerate fibers have a high surface to volume ratio that supports high volumetric ethylene production. These multifunctional systems are fabricated in one step by employing additive manufacturing approaches that take advantage of co-extrusion of the catalyst and membrane. This approach allows the team to make thin membranes interspersed between thicker, more porous catalyst layers that facilitate the reaction between oxygen and methane, and more broadly to tune the transport and reaction rates so that these match each other.
The thinner membranes increase the rate at which surface oxygen reacts with methane, which ramps up ethylene production but could also allow for more undesired oxygen to accumulate and react with ethylene. To get around this problem, the team changed the number of OCM-active sites in their reactor such that methane-oxygen reaction rates precisely matched the oxygen diffusion rate. That change causes all the oxygen introduced into the system to be used up in reactions with methane before it has the chance to see ethylene.
“Tuning the methane activation rate to the oxygen diffusion rate gave unexpectedly high ethylene yields compared to a conventional reactor composed of the same catalyst material,” said James Wortman, a doctoral student of chemical engineering working in Suljo Linic’s lab.
Being able to easily tune the properties of both membrane and catalyst layers could offer a promising way to optimize reactors for other desired chemistries, such as fuel cells, electrolyzers, and partial oxidation reactions, said Wortman.
The research is funded by the US DOE Office of Basic Energy Sciences, Division of Chemical Sciences and the National Science Foundation Graduate Research Fellowship.