Research Programs for Undergraduates
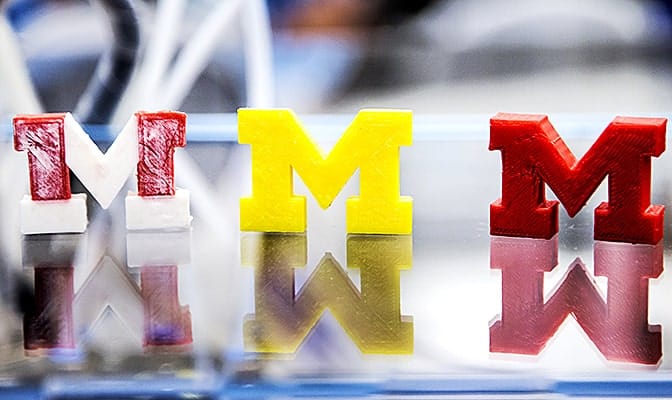
For U-M Students
Summer Undergraduate Research in Engineering (SURE)
For U-M undergraduate students, SURE offers summer research internships to outstanding undergraduate students who have completed at least their first year by the time of the internship (rising first years through seniors). Participants have the opportunity to conduct 10-12 weeks of full-time summer research with some of the country’s leading faculty in a wide range of engineering disciplines. The program provides opportunities for students to assess their interests and potential in pursuing research at the Masters or PhD level in graduate school. All participants must apply online through the SURE website. Accepted applicants from the University of Michigan receive guidance from a faculty advisor in a College of Engineering research facility, a stipend of $6,000, attend regular meetings and seminars, and submit an abstract or other designated assignment to showcase their summer research experience. Learn more about SURE.
Please note:
- Chemical Engineering does not require any additional documents other than a resume.
- Chemical Engineering faculty each develop their own processes for selecting candidates to join their labs. As such, faculty may contact candidates as part of their decision-making process.
Undergraduate Research Opportunity Program (UROP)
The Undergraduate Research Opportunity Program (UROP) creates research partnerships between first and second year students, and faculty, research scientist, and staff from across the University of Michigan community. All schools and colleges are active participants in UROP,thereby providing a wealth of research topics from which a student can choose. Begun in 1988 with 14 student/faculty partnerships, today, approximately 1200 students and over 700 faculty researchers are engaged in research partnerships. Learn more about UROP.
For Undergrads At Other Universities
Summer Research Opportunity Program (SROP)
For non-U-M undergraduate students, SROP offers summer research internships to outstanding undergraduate students who have entered or completed their junior year by the time of their internship. Participants have the opportunity to conduct seven to eight weeks of full-time summer research with some of the country’s leading faculty in a wide range of engineering disciplines. The program provides opportunities for students to assess their interests and potential in pursuing research at the PhD level in graduate school. All participants must apply online through the SROP website. Accepted applicants receive guidance by a faculty advisor in a College of Engineering research facility, a stipend of $4,500, room and some board, travel allowance, GRE Test Prep, weekly seminars and workshops, produce a short YouTube video of their summer research project and/or experience, and potential attendance at a Big Ten CIC Research Symposium. Learn more about SROP.
For department information, contact Colette Sherfey, ChE Graduate Programs Manager.
SURE/SROP Projects
The application deadline is January 8, 2025. We will contact you when the list has been updated with instructions for our internal ChE-specific selection process.
All participants must apply online through the SURE website.
ChE Project #1: Dynamic Electrochemical Systems via an Electromagnetically Reconfigurable Electrode-Electrolyte Interface
Faculty Mentor: Albert Liu, [email protected]
Project Description: The pressing need to reach net-zero emissions drives a global effort to de-carbonize the chemical sector. One of the most promising strategies is to electrify the chemical production processes and establish distributed manufacturing with lower energy and chemical footprint. Building nature-inspired synthetic electrochemical systems that simultaneously possess high activity, selectivity, recyclability and adaptability represents an important step towards sustainable and responsible production of value-added chemicals. By bringing together recent advances in conductive microgel synthesis and field-assisted self-assembly of colloidal microparticles, this proposed study aims to develop a colloidal microgel based surface modification strategy to dynamically reconfigure the electrode–electrolyte interfaces in electrocatalytic systems, in order to enable temporally and spatially resolved control of reaction pathways and product selectivity in next-generation adaptive electrochemical systems.
ChE Project #2: Nanopore Electroporation for Exosomal Uptake and Delivery of Therapeutic Molecules Towards Rapid, On-Demand Treatment of Spinal Cord Injury
Faculty Mentor: Albert Liu, [email protected]
Project Description: Effective treatment of acute spinal cord injuries (SCI) constitutes a gap in the triumphs of modern medicine. SCIs are caused by violent events, such as car crashes, and structural degeneration from aging, a challenge that will grow in the coming years with the expanding global elderly population. Rapid, initial treatment is key in SCI prognosis: around 20% of SCI patients treated in less than 24 hours made meaningful recoveries, as compared to only 9% treated later (in North America). The general SCI treatment strategy involves two steps: neuroprotection and neuroregeneration, with the initial neuroprotective treatment focusing on mitigating further injury from a traumatic immune response. After intense immune activity abates (~14 days), mesenchymal stem cell (MSC) transplants are used to rebuild nerve function, and they are considered state of the art for neuroregeneration. Both treatment steps commonly involve surgical procedures, which necessitates access to specialized centers and skilled surgeons. MSC therapies also suffer from high immune activity harming MSC viability, imprecise delivery (MSC migration/trapping), and the necessity for multiple transplants. The proposed study aims to bridge this service deficiency by developing novel neuroprotective and neuroregenerative therapeutics for on-demand SCI treatment using engineered exosomes.
ChE Project #3: Harnessing the Hydrovoltaic Effect for Sustainable Energy Harvesting: From Fundamentals to Real-World Applications
Faculty Mentor: Albert Liu, [email protected]
Project Description: Water is the Earth’s most abundant and versatile energy resource, yet its vast energy potential remains largely untapped. Covering 71% of the Earth’s surface, water consumes about 35% of the solar energy received by the Earth, corresponding to a remarkable 60 petawatts (1015 W). If just a small portion of the tremendous energy contained in water could be harvested, it would readily satisfy the global energy demand of 18 terawatts (1012 W). The hydrovoltaic effect, a recently discovered phenomenon that converts the latent energy in water into electricity, holds the promise of introducing new ways we can extract energy from the ambient environment. Even though many practical hydrovoltaic energy harvesting devices have been demonstrated in the last few years, a comprehensive theoretical framework on the structure-property-relationship between the network structure of the underlying nano-materials and the resulted power outputs is still lacking. By investigating the interaction between water and carbon nanostructures, such as nanoflakes and nanotubes, we aim to unlock the full potential of hydrovoltaic-based energy harvesting.
ChE Project #4: Dynamic Nanopore Microfluidic Transfection via Electroporation
Faculty Mentor: Sasha Cai Lesher-Perez, [email protected]
Project Description: Objective and Methods: Electroporation is a transfection method used to deliver macromolecules such as DNA, mRNA, and proteins into cells. Transfection has a variety of medical applications including cellular manipulation, therapeutic effects, and stem cell differentiation. Cell based therapies including wound healing with patient-derived stem cells would benefit from an advanced transfection technology to increase throughput and efficiency. We are developing a new tool that builds upon electroporation called Dynamic Nanopore Microfluidic Transfection via Electroporation (DyNaMiTE). DyNaMiTE is a technology that will leverage nanopore electroporation in a microfluidic device. In nanopore electroporation, an applied electric field is localized at the nanopores embedded in an insulated membrane to provide a safer alternative of transfecting cells.
Responsibilities: The student’s responsibilities in this project will be designing microfluidic device molds using 3D modeling software (i.e., Fusion360), designing a clamping mechanism to secure the final microfluidic device, and fabricating microfluidic devices. If the student is interested in cell work, they would also can learn how to culture cells and electroporate cells. This student will develop skills in CAD software, generating microfluidic devices, operating/navigating various 3D printing platforms, microscopy, operating electrical equipment (oscilloscope, waveform generators, amplifiers, etc.) and cell culture. The student will grow in knowledge about the biomedical applications this technology possesses in reference to transfecting cells with macromolecules.
ChE Project #5: Modeling microfluidic flow within granular systems
Faculty Mentor: Sasha Cai Lesher-Perez, [email protected]
Project Description: Objective and Methods: Microfluidic organ-on-chip models aim to more closely mimic complex tissue environments. In pursuit of mimicking3Dtissue microenvironments, we combine granular microgels with dynamic flow in a microfluidic configuration. This granular systemin ables perfusion through void spaces between each microgel which increases mass transfer rates to increase nutrient and oxygen supply while increasing waste removal rates. The granular scaffold also provides a similar mechanical environment to tissue while individual microgels provide a similar biochemical environment. Development of a COMSOL model will help better understand physical effects of fluid flow through the system. With this new perspective, the aim of the project is to improve feeding rates, waste removal, and flow impacts for better outcomes.
Responsibilities: Student will primarily work on translating experimental parameters to the development of a COMSOL model. This work will require the use of COMSOL Multiphysics. Basic understanding of fluids will help but is not needed.
ChE Project #6: Computational studies of self-assembly at the nanoscale
Faculty Mentor: Sharon Glotzer, [email protected]
Prerequisites: Some thermodynamics is helpful but not strictly required.
Project Description: Assist in computational studies of molecular dynamics and Monte Carlo simulations to explore fundamental properties of self-assembly at the nanoscale. Projects are varied and students will be matched with researchers based on skills, interests, and current timeline of the projects. Simulations are run on high-performance computing clusters at UM and national resources such as NSF ACCESS. Students will learn to run simulations, analyze results, and use a formal framework for managing data. Some knowledge of thermodynamics and statistical mechanics is helpful but not strictly required.
ChE Project #7: Developing tools in Python for simulations, analyses and data management of soft matter simulations
Faculty Mentor: Sharon Glotzer, [email protected]
Prerequisites: Moderate proficiency in either Python or C++.
Project Description: Assist in the development of open-source software packages for nanoparticle and soft matter simulations in use by many research groups inside and outside of the University of Michigan. In this project, you will learn standard molecular dynamics and Monte Carlo particle simulation techniques and grow your professional software development skills. Software packages are written using Python, C++, so basic prior knowledge of at least one of these is required. Prior Python users will have the opportunity to learn C++ if they wish, and vice versa.
ChE Project #8: Polyelectrolyte Simulations
Faculty Mentor: Ron Larson, [email protected]
Project Description: Polyelectrolytes (PEs) widely exist in life, such as DNA/RNA, and they have many applications in fields such as drug delivery, sewage treatment. However, we do not understand most of the aspects of how PE molecules behave, not only because there are too many variables controlling the PE phenomena, but also PE system is strongly correlated due to electrostatic interaction, dipole-dipole interaction, hydrogen bonding, and so on. Here we are interested in understanding the fundamentals of PE complexes using via all-atom or coarse-grained molecular dynamics simulations. The project will involve learning the molecular dynamics software package GROMACS, and directly simulating complex formation by oppositely charged polyelectrolytes. The results will be compared to experiments and theory.
ChE Project #9: Polyelectrolyte phase behavior and rheology
Faculty Mentor: Ron Larson, [email protected]
Project Description: Polyelectrolytes (PEs) widely exist in life, such as DNA/RNA, and they have many applications in fields such as drug delivery, underwater adhesives, food additives and others. When polyelectrolytes of opposite charge are mixed, they form complexes or “coacervates” with unusual flow properties, including high viscosity and gel-like structure important for their applications, and important in the structure of biological cells. The project is to mix well-defined polyelectrolyte and measure their properties experimentally, especially their flow properties using a mechanical rheometer. The work will be guided by a Ph.D. student and will train the student not only in polyelectrolyte physics and technology, but also in the measurement of properties of viscous solutions and gels.
ChE Project #10: Multicomponent aerogels for solar thermal applications
Faculty Mentor: Andrej Lenert, [email protected]
Prerequisites: Experience with MATLAB or similar
Project Description: Silica aerogels are an exciting alternative to conventional solar thermal collectors for use in concentrated solar power. They are one of the most thermally-insulating materials and they can also be optically transparent. Our goal is to develop a facile synthesis procedure that improves their properties by coating them with a second material: one that is capable of suppressing infrared radiative losses while also maintaining high optical transparency. This project will provide experience in wet lab chemistry and material characterization, and also provide knowledge in conductive and radiative heat transfer.
ChE Project #11: Controlled synthesis of high surface area nonstoichiometric mixed metal oxides for hydrogen generation from water
Faculty Mentor: Eranda Nikolla, [email protected]
Project Description: Non-stoichiometric mixed metal oxides (e.g., perovskites, double perovskites, Ruddlesden-Popper (R-P) oxides…) are redox and thermally stable materials largely characterized by the oxygen non-stoichiometry in their structure. The latter is a key factor in providing excellent ionic conductivity to this family of materials, enabling their application as promising heterogeneous catalysts for a number of relevant reactions to energy and chemical conversion and storage. One application of these oxides is that of catalyzing oxygen electrochemical reaction related to H2 generation from water. Electrolysis of water provides a sustainable pathway toward storing electrical energy from renewable sources in the form of chemical bonds. The objective in this project is to use reverse microemulsion and hydrothermal syntheses to control the surface area and nanostructure of nonstoichiometric mixed metal oxides for effectively catalyzing the electrochemical reactions involving oxygen. Previous experience in a wet lab is preferred.
ChE Project #12: Efficacy of Antibody Drug Conjugates for Cancer in Tumor Organoids
Faculty Mentor: Greg Thurber, [email protected]
Project Description: Antibody Drug Conjugates (ADCs) are a rapidly growing class of cancer therapeutics that target specific small molecule drugs to cells by binding to receptors expressed on the surface of cancer cells. The development of these agents is complex due to the need for designing the monoclonal antibody, small molecule payload, and linker connecting the two. Importantly, interactions with the immune system can also occur due to binding of the Fc domain of antibodies to immune cells. This project will look at the delivery of ADCs in tumor organoids consisting of a mixture of cancer cells and immune cells grown in 3D culture with the goal of understanding and predicting the distribution in this complex environment.