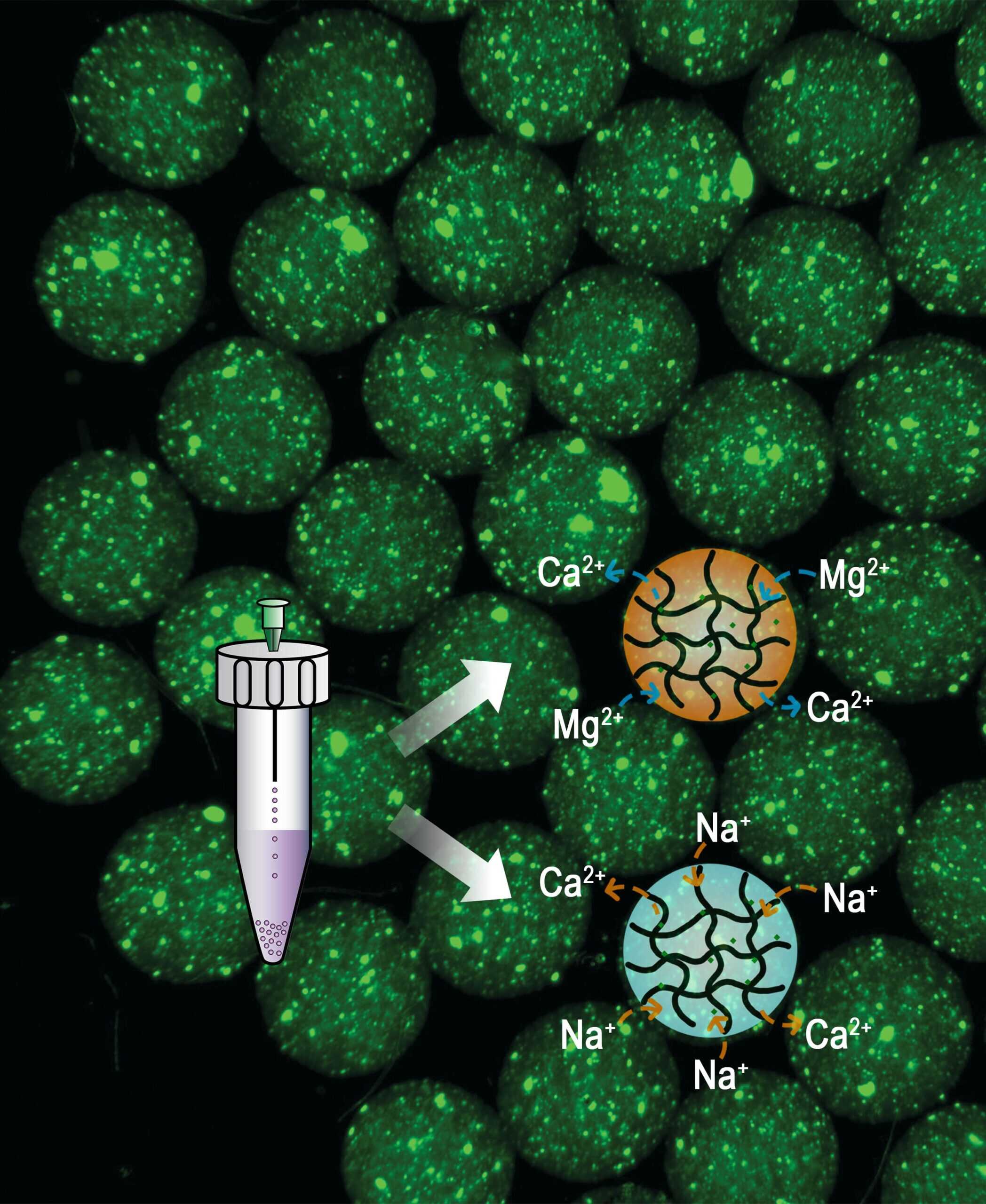
A simpler way to make microgels for programmable drug release
Dunking a calcium alginate microgel in ion baths helps finely tune drug release profiles without complex equipment, opening up microgel synthesis to more researchers.
Dunking a calcium alginate microgel in ion baths helps finely tune drug release profiles without complex equipment, opening up microgel synthesis to more researchers.
Written by Patricia DeLacey
A new straightforward way to make an injectable gel capable of releasing multiple drugs at specific speeds improves scalability and access to the technique, according to a University of Michigan study.
Made of natural or synthetic polymers with a high water content, hydrogel microparticles—called microgels—measure about 1 to 100 micrometers wide and encapsulate even smaller drug particles.
By tuning microgel properties such as particle size, swelling behavior or the degree to which gel molecules are cross-linked, researchers can precisely control the release of encapsulated drugs—known as programmable delivery.
“A microgel suspension behaves in part like a solid with the controlled delivery and in part like a liquid with its flexibility, making it an ideal form for injections,” said Albert Liu, an assistant professor of chemical engineering, macromolecular science and engineering and materials science and engineering at U-M and corresponding author of the study.
A single injection containing multiple timed drug releases helps minimize invasiveness during complex medical treatments. In certain cancer treatments, one microgel injection could deliver a fast-acting drug to destroy cancer cells, a sustained-release drug to inhibit blood vessel development around a tumor and a delayed-release drug to combat side effects or tumor responses.
Up to this point, microgels were made by carefully developing covalent bonds—two atoms that share electrons—to precisely time drug delivery. While finely controlled, the complex approach requires specialized chemicals and equipment, making it difficult for industries or smaller academic laboratories to manufacture them.
The research team developed a simpler, alternative strategy to make injectable microgels using ionic bonds—interactions between charged ions that are easier to break than covalent bonds.
“We can confidently say this is one of the simplest ways to synthesize microgels with high reproducibility. All you need is an adequate sized tube, a needle and access to a centrifuge,” said Sungwan Park, a doctoral student of chemical engineering at U-M and co-author of the study.
The process first combines alginate—a naturally occurring carbohydrate in brown algae like kelp—with calcium to form a microgel. After synthesis, treating the microgel in baths of different ions like magnesium or sodium replaces the tight bond between calcium and alginate with a bond that is easier to break.
Adjusting factors like the duration of ion bath incubation and the concentration of the ion bath precisely dials in the ratio of calcium to magnesium or sodium ions within the resulting microgels. This dictates the mesh size of the polymer gel, which controls the drug release rate. By mixing and matching different post-synthesized ionic exchanged microgels into larger gel capsules, several pre-programmed release profiles can be accomplished at once.
Powerful microscopy techniques helped characterize microgel physical properties that impact drug release times like the surface roughness, the evenness of ion distribution or swelling behavior.
“The parameter space is immense and I’m sure we can continue to optimize the method further. For instance, different factors that haven’t been tested, like the alginate concentration or how fast we spin the tube, could also be used to finely adjust microgel properties,” said Jihpeng Sun, a doctoral student of chemical engineering at U-M and co-lead author of the study.
The research team demonstrates drug release profiles by encapsulating brightly colored test drugs within the microgel for easy tracking. Exploring microgels with both a single drug and a mixture of drugs, the study showcases how varying ion-exchange treatments and drug cargoes achieve programmable release patterns.
“We hope the simplicity of this method can help anyone interested in controlled drug delivery pick up and execute this method without needing to purchase expensive equipment or spend time troubleshooting,” said Liu.
Fiona Nikolla—a high school student at the Gene L. Klida Academy for International Studies in Sterling Heights, Michigan—also contributed to this study.
The research teams thanks the Michigan Materials Research Institute (MMRI) and the Michigan Center for Materials Characterization through NSF grant (DMR-1625671), which supported the SEM resources used in this work
This research was funded by the National Science Foundation Center for Complex Particle Particle Systems (2243104) and the American Chemical Society Petroleum Research Fund (66979-DNI10).
The team has applied for patent protection with the assistance of U-M Innovation Partnerships.
Full citation: “Programmable cargo release from jet-printed microgel particles via an in situ ionic exchange method,” Rong Ma, Jihpeng Sun, Sungwan Park, Fiona Nikolla, Albert Tianxiang Liu, Chem and Bio Engineering (2025). DOI: 10.1021/cbe.5c00017