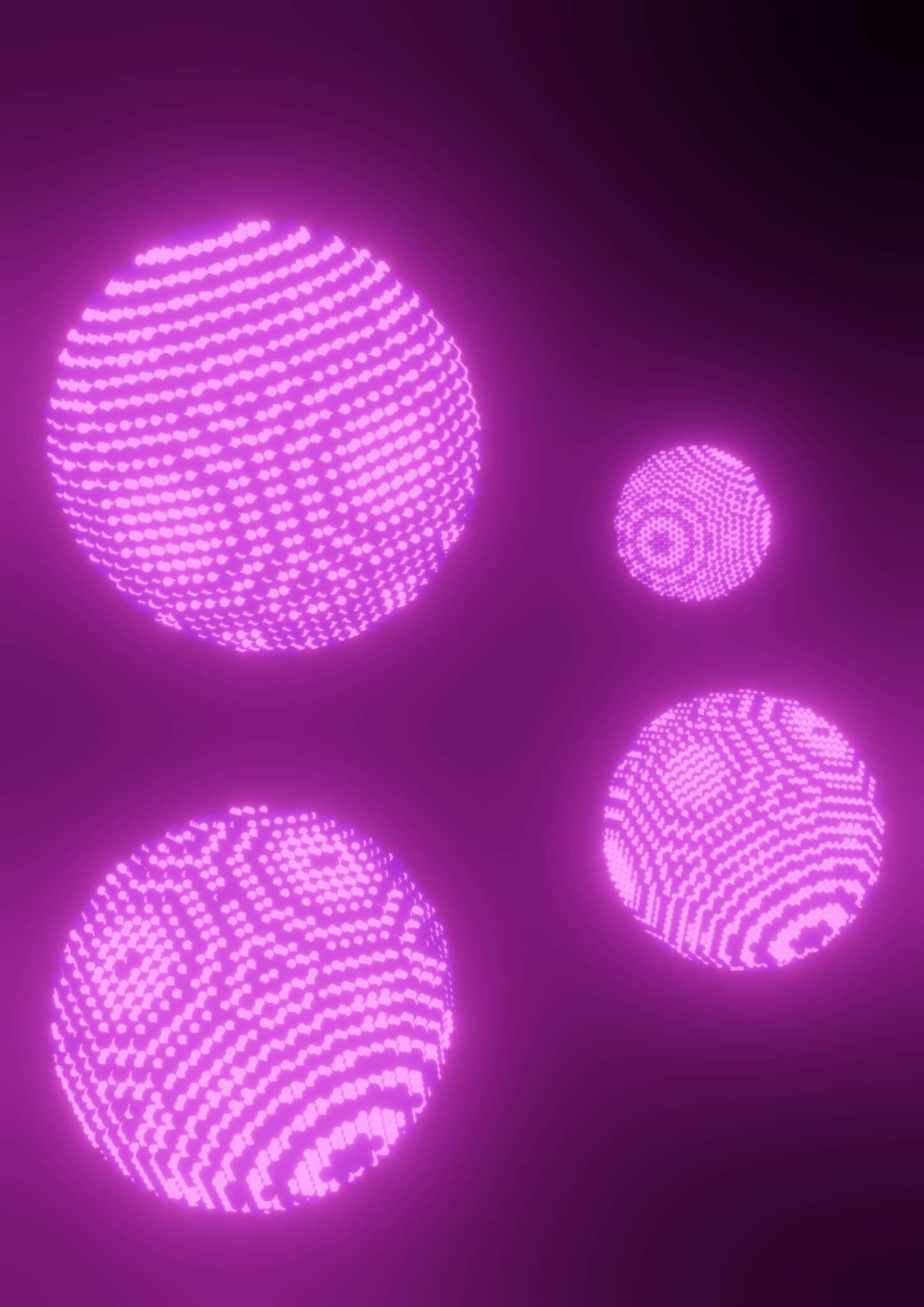
Scientists observe composite superstructure growth from nanocrystals in real time
The findings could enable engineers to more reliably manufacture next-gen materials by combining different nanocrystals.
The findings could enable engineers to more reliably manufacture next-gen materials by combining different nanocrystals.
Written by Derek Smith and originally published by Michigan Engineering.
For the first time, scientists and engineers have observed in real time how two types of nanoparticles made from different materials combine into new composite materials. The findings, reported by a team led by the University of Pennsylvania and University of Michigan, could help engineers have more control over the assembly of materials that combine the desirable properties of each particle—such as photoluminescence, magnetism and the ability to conduct electricity.
“We are designing new materials that combine different kinds of functions in ways that are not possible with the materials we have today,” said Sharon Glotzer, the Anthony C. Lembke Department Chair of Chemical Engineering at the University of Michigan and co-corresponding author of the study published in Nature Synthesis.
The composite structures are a type of binary nanocrystal superlattice and could be used for electronic devices, optical devices, and energy production and storage.
“Combining photoluminescent and magnetic nanoparticles, for example, could allow you to change the color of a laser using a magnetic field,” said Emanuele Marino, a co-first author of the paper and a former postdoctoral researcher at the University of Pennsylvania.
Engineers typically create binary nanocrystal superlattices by mixing nanoparticle building blocks in a solution and letting a droplet of the solution dry out. As the droplet shrinks, the particles combine into the desired superstructures. Engineers then hit the crystals with X-rays to see the resulting nanocrystal structures. Each crystal structure scatters X-rays in a unique pattern, which serves as a fingerprint to identify the crystals.
Seeing how those crystals assemble in real-time has been a scientific challenge because they form too fast for most X-ray scattering techniques. Without seeing steps leading up to the final structure, scientists are left guessing how their nanocrystal mixtures lead to superstructures.
“Figuring out how these materials react with one another will allow us to build a more comprehensive library of the structures they can form when they combine,” said Christopher Murray, the Richard Perry University Professor of Chemistry at the University of Pennsylvania and co-corresponding author of the study.
The team created the first real-time X-ray scattering measurements of the superlattices by slowing down the assembly process and using faster X-ray scattering techniques with the help of the National Synchrotron Light Source II at Brookhaven National Laboratory in Upton, New York.
“The facility’s high X-ray flux and rapid data collection could keep up with the speeds at which the crystals formed,” said Esther Tsai, a staff scientist at the Brookhaven National Laboratory and study co-author.
To slow lattice assembly, the researchers mixed different nanoparticles into an oil emulsion – almost like a magnetic salad dressing – then placed the emulsion in water. The nanoparticle mixture shrank as the oil diffused into the water, but much more slowly compared to the conventional air-drying method. After an initial, rapid growth phase that lasts up to five minutes, the nanocrystals come together by slowly expelling the last of the remaining oil over three to five hours.
Getting eyes on the nascent crystals allowed the University of Michigan team to derive the physics explaining how the lattices formed, modeling the process with computer simulations.
“With temporal information from experiments, we can construct a predictive model that reproduces not just the final structure, but the structure’s entire assembly pathway,” said Sharon Glotzer, the Anthony C Lembke Department Chair of Chemical Engineering at the University of Michigan and co-corresponding author of the study.
The team discovered that binary nanocrystal superlattice assembly occurs through short-range attractions between the nanoparticle building blocks, regardless of the type of nanoparticle used, and “further confirmed that no intermediate phases formed before the final crystal, and the surface of the emulsion droplets did not play a role in forming the crystal,” said Allen LaCour, a former doctoral student of chemical engineering at the University of Michigan and co-first author of the study.
Without other explanatory factors, the simulations concluded that the strength of the nanocrystal interactions is the primary factor that determines superlattice structure in the shrinking droplets. The interaction strength can be changed with the particles’ size and electric charge, or adding certain elements to the particles. The U-M team’s computer models can simulate the impacts of those changes.
The National Science Foundation, the Office of Naval Research, and the National Recovery and Resilience Plan supported the research. Emanuele Marino is now an assistant professor at the University of Palermo in Italy. Allen LaCour is now a postdoctoral researcher at Lawrence Berkeley National Laboratory. Sharon Glotzer is also the John Werner Cahn Distinguished University Professor of Engineering and Stuart W. Churchill Collegiate Professor of Chemical Engineering.