Undergraduate Research Projects
The following pages describe undergraduate research projects available in the chemical engineering department for pay, credit or as a volunteer during the Winter 2024 term. Visit the Faculty Labs page for more information on obtaining research positions.
Professor Lola Eniola-Adefeso
Characterizing activation of neutrophils interacting with particle-based therapeutics
This project will expand our knowledge of physiological mechanisms by which polymeric particles function in reprogramming innate immune cells, specifically neutrophils. In recent work, the Eniola lab has identified an optimized degradable material for particle-based inflammation modulation. Salicylic acid, the active ingredient in aspirin, can be polymerized (PolyAspirin or Poly-A) and formulated into microparticles. Poly-A particles effectively reduce neutrophil accumulation in localized inflammation while also providing anti-inflammatory benefits as it degrades, releasing salicylic acid. Thus far, our knowledge is limited in understanding the mechanism of action regarding Poly-A particles and interactions with immune cells. Our work focuses on understanding Poly-A interactions with neutrophils, phagocytic white blood cells that reside in the blood. Neutrophils have several mechanisms to respond to inflammation including cytokine production, the release of reactive oxygen species (ROS), and neutrophil extracellular trap (NET) formation. The goal of this project is to develop and utilize an assay to evaluate ROS release in neutrophils when treated with polymeric particles of interest. The student selected for this project would first aid in assay design alongside a graduate student, then would gain hands-on research experience working with human neutrophils.
Evaluating polymer molecular weight as a parameter in tuning interactions between polymeric particles and neutrophil interactions
Poly(lactic-co-glycolic acid), also known as PLGA, is a degradable polymeric material used clinically in drug delivery. Recently, the Eniola lab has found that polymeric particle degradation can be utilized as an anti-inflammatory property to modulate circulating phagocytic white blood cells. In this project, an undergraduate will be responsible for fabricating and characterizing PLGA particles from various molecular-weight polymers. The characterization will include scanning electron microscopy (SEM), dynamic light scattering (DLS), and degradation. Further work on this project may include testing particles with in vitro assays to investigate the effects of molecular weight on particle functionality and interactions with immune cells.
Investigating density as a property to enhance localization of vascular targeted carriers
Cardiovascular diseases (CVDs) account for 1 in 5 deaths, in pre-covid years. These CVDs, such as atherosclerosis result from plaque formation in the arteries, which leads to blood clot formation. Vascular targeted carriers (VTCs) – biocompatible vehicles which carry drugs to the site of interest – have shown potential in the treatment of CVD. However, current research has been limited by poor adhesion of VTCs to the endothelium due to their interaction with red blood cells, and other blood components. Preliminary results have shown enhanced margination and localization of VTCs to the endothelium with increasing particle density. To limit external contributions arising from differences in the VTC materials (polystyrene, titania, and silica) used in previous work, we will carry out a further investigation by varying the density of the gold-loaded VTCs by varying the amount of gold loaded into the hydrogel matrix. For this project, an undergraduate student
would carry out particle fabrication/characterization and assist with in vitro studies upon training.
Professor Xiwen Gong
Design New Hybrid Nanomaterials for Wearable Medical Devices
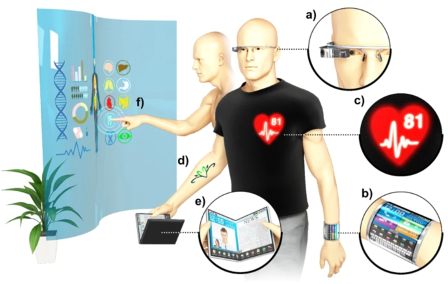
An aging population and increasing health expenditure demand for the next generation of medical devices that offer comfort, convenience, and accurate detection of body signals. Generation of flexible devices such as those shown here is the goal of the Gong Lab. Flexible medical devices offer the comfort of stretchability over the skin and the convenience of placement on anywhere on the body. This research project develops soft electronic materials that will emit light in the near infrared region (750 – 2500 nm) to detect body signals. Several substances in the human body including glucose and oxygen absorb in the infrared spectrum of light allowing us to detect these substances when infrared light is shined on the body. Students will work closely with graduate students within the lab to develop a wearable near infrared LED based bioelectronics that will offer ease of medical care. No prior experience in optoelectronics is required. Interested students will gain a strong foundation in material and optoelectronic synthesis and characterization.
For more information, visit the Gong Lab website.
Professor Jinsang Kim
Purely organic phosphors for display and sensors
Phosphorescent materials are enhancing and broadening the usefulness of organic compounds in a wide variety of applications including OLED, photovoltaics, and sensors. OLEDs and solid-state lighting (SSL) research in both academic and commercial settings are driven by the promise of highly efficient devices manufactured via economic and versatile processes. Of the emissive materials employed in OLEDs and SSL, phosphorescent compounds produce much higher efficiency devices than those based on fluorescent emitters by utilizing spin-parallel electrons and emitting photons from the decay of triplets. Organo-metallic compounds are thus often doped into organic hosts to impart a phosphorescent pathway into otherwise triplet-forbidding carbon-based materials. However, phosphorescence from metal free, purely organic, compounds is almost always either strictly forbidden or only extremely weakly allowed, leaving pure organics undesirable for useful applications. We developed a novel material system, metal-free purely organic phosphors recently and demonstrated. Directed intermolecular heavy atom effects are uniquely implemented in aromatic carbonyl molecules to promote spin-orbit coupling and suppress vibrational dissipation. Color tuning by rational molecular design, highly sensitive optical sensors, and PhOLED are the research topics for development.
Self-signal Amplifying Molecular Biosensors
We have been devising molecular sensors having a dual-signaling capability: visible color change and fluorescence emission. The capability to generate a visible color change upon recognition of a target analytes will allow equipment-free detection. Fluorescence emission signaling upon detection is designed to provide a high sensitivity by means of signal amplification via the fluorescence resonance energy transfer (FRET). The sensory molecules are composed of a receptor, a diacetylene moiety, and a fluorophore. The molecules are devised to self-assembled to form a liposome followed by topochemical polymerization of the diacetylene moiety. The receptor will undergo a shape change upon recognition of a target analyte. This shape change will turn on the mechanochroism of the polydiacetylene unit of the liposome and produce a color change and develop fluorescence emission. Once the fluorescence emission is turned on the fluorescence energy of the fluorophore will amplify the turned-on signal through FRET. This research project has various interesting aspects: molecular design, self-assembly, sensor physics, and device fabrication.
Professor Nicholas Kotov
Self-assembly of Nanoparticles; Chiral Nanomaterials (Chiral catalysis, origin of homochirality on Earth); Hedgehog Particles; Biomimetic Nanocomposites
Visit the Kotov Lab website for additional details.
Professor Mark J. Kushner
Computational Plasma Science and Engineering Group (CPSEG)
Our research group computationally investigates how plasmas (partially ionized gases) activate chemistry for materials processing, energy and healthcare applications. A typical undergraduate research project might include, for example, aiding in the development and use of computer models to investigate how plasma activated liquids beneficially interact with tissue, or how reactive fluxes to surfaces can uniquely craft nano-scale electronics.
Atmospheric Pressure Plasmas for Catalysis
Atmospheric pressure plasmas are able to activate gases by creating radicals and ions at low gas and materials temperatures. An active area of research is plasma-catalysis, using this ability to efficiently produce radicals to speed the rate of processing of catalysts and improve their selectivity. These processes are being investigated to produce “solar fuels” – using solar generated electricity to produce plasmas, which convert CO2 into high value fuels and hydrocarbon feedstocks. The research project will involve a computational investigation of reactor configurations and strategies to address chemical conversion in plasma catalysis.
Professor Joerg Lahann
Protein-based Gene Delivery, Therapeutic, and Contrast Agent Nanoparticles
Synthetic protein nanoparticles (SPNPs) are generated through a process known as electrohydrodynamic jetting, primarily, or through desolvation in solution.
This interdisciplinary research project consists of multiple thrusts: [1] determination of factors dictating structure-function relationships; [2] gene delivery through mRNA or pDNA; [3] delivery of targeted small molecule therapeutics; [3] investigation on the uptake mechanisms for nanoparticles into cells; [4] investigation into the mechanisms of endosomal escape after uptake; [5] novel contrast agents for theranostic applications.
We will utilize an electrified jetting process to produce monophasic or polyphasic nanoparticles. In this process, we apply high electrical potential on a jetting liquid to generate a cone and jet. At certain experimental conditions, this liquid jet is accelerated and eventually breaks down to form solid particles with diameter in nanometer scale. We will utilize several materials for this process and characterize the resulting nanoparticles with electron microscopes (SEM and TEM) and size/charge analysis (DLS). The produced nanoparticles will be used for the study a variety of genetic or therapeutic payloads to cells (confocal microscopy and flow cytometry). The possible applications of these studies are targeted drug delivery, cell imaging, and the development of gene therapies for immunization or the correction of genetic disorders.
Biomedical Scaffolds for Regenerative Medicine Research Applications
The main objective of these projects is the development of scaffolds that are capable of directing cell, tissue, and organoid growth in and on a substrate that mimics in-body conditions.
This is primarily achieved through the use of fibronectin and other extracellular membrane proteins which are shear assembled onto a bioprinted lattice. The major thrusts of this research are [1] organoid development; [2] high throughput scaffold production; [3] composite systems with a plurality of biomacromolecules; [4] micromechanically induced tension and deformation in the scaffolds; [5] post-production modification of scaffolds for enhanced biointeractions.
We will utilize a 3d bioprinter to develop lattices appropriate for protein deposition. A protein solution is then applied a variety of shear rates to the lattice, utilizing multiple geometries to impact the final alignment of the network that is formed. The process of network formation results in fibrilization and growth in various degrees, directions, and densities. We will utilize several materials for this process and characterize the resulting scaffolds with optical microscopies, micromechanical methods, and bioavailability assays. The produced scaffolds will be used for the study a variety of cell, tissue, and organoid growth studies, to include stem cell differentiation. Key methods will include cell room protocols, cell differentiation analysis, viability/toxicity, and cellular alignment. The possible applications of these studies are the large scale production of scaffolds for pre-clinical and translational medicine studies, tissue and organ regeneration, and stem cell-based therapies.
Chemical Vapor Polymerization (CVP) of Liquid-Crystal Templated Nanofibers and Area Selective Deposition Systems.
Broadly, this work is focused on the usage of a chemical vapor deposition (CVD) instrument to induce vapor phase activation of functional poly(para-xylylene)s (PPX) with subsequent polymerization on deposition.
This interdisciplinary research project consists of multiple thrusts: [1] use of various liquid crystals to direct nanofiber growth that results in nanocomplex surfaces with unique optical, chemical, physical, and biological properties; [2] use of various substrates and engineering controls to direct polymerization to only occur on specified regions of the substrate that results in controlled functionalization on the nano- and micro-scales.
We will utilize an CVD to produce reactive PPX-based monomers. In this process, we apply high heat and high vacuum to target a substrate in a cooled reaction chamber. At certain experimental conditions, we can direct polymer formation into nanofibers that adopt conformations and properties that are directed by the LC templates. This results in highly regular nanoscale feature that can express chirality, curling, twisting, linearity, or networking based on LC type and reaction conditions. Additionally, we have discovered that PPX based monomers can be guided to polymerize on specific features of a multimaterial substrate, resulting in bottom-up polymeric features that can be directed precisely. We will utilize techniques to assess these systems: atomic force microscopy, photoluminescence spectroscopy, electron microscopy, surface reaction assays, surface energy assessment, and cell growth assessment. The possible applications of these studies are antifouling, antibiofouling, directed cell growth, unique barrier coatings, nanophotonics, and the structuring of polymeric materials at microchip feature scales.
Professor Ronald Larson
Polyelectrolyte phase behavior and rheology
Polyelectrolytes (PEs) widely exist in life, such as DNA/RNA, and they have many applications in fields such as drug delivery, underwater adhesives, food additives and others. When polyelectrolytes of opposite charge are mixed, they form complexes or “coacervates” with unusual flow properties, including high viscosity and gel-like structure important for their applications, and important in the structure of biological cells. The project is to mix well-defined polyelectrolyte and measure their properties experimentally, especially their flow properties using a mechanical rheometer. The work will be guided by a Ph.D. student and will train the student not only in polyelectrolyte physics and technology, but also in the measurement of properties of viscous solutions and gels.
Polyelectrolyte Simulations
Polyelectrolytes (PEs) widely exist in life, such as DNA/RNA, and they have many applications in fields such as drug delivery, sewage treatment. However, we do not understand most of the aspects of how PE molecules behave, not only because there are too many variables controlling the PE phenomena, but also PE system is strongly correlated due to electrostatic interaction, dipole-dipole interaction, hydrogen bonding, and so on. Here we are interested in understanding the fundamentals of PE complexes using via all-atom or coarse-grained molecular dynamics simulations. The project will involve learning the molecular dynamics software package GROMACS, and directly simulating complex formation by oppositely charged polyelectrolytes. The results will be compared to experiments and theory.
Professor Xiaoxia (Nina) Lin
Engineering of synthetic microbial consortia for sustainable biofuel/bioproduct manufacturing
Microbes are everywhere in nature and they live in diverse communities that show remarkable metabolic capabilities and robustness. Inspired by these synergistic ecosystems, our lab has been developing a new approach for microbial engineering and biochemical production – design and construction of synthetic microbial consortia consisting of different specialists to accomplish a complicated task. One application focus has been synthesis of fuels and chemicals from lignocellulosic biomass. Our designed consortium (see figure) includes a cellulolytic member responsible for hydrolyzing hemicellulose and cellulose (main components of lignocellulosic biomass) into mono and oligosaccharides and a fermenting member for converting mono and oligosaccharides into desired molecules such as isobutanol, an advanced biofuel. Such a synthetic microbial consortium integrating saccharification and fermentation capabilities will enable one-step “consolidated” bioprocessing (CBP), a potential breakthrough technology that can lead to cost-effective production of lignocellulosic biochemicals. The general framework of engineering defined co-cultures of coordinated specialists could offer exciting new opportunities for the efficient and flexible production of many valuable chemicals from other non-conventional bio-feedstocks.
Understanding and engineering microbiomes using microfluidic droplet technology
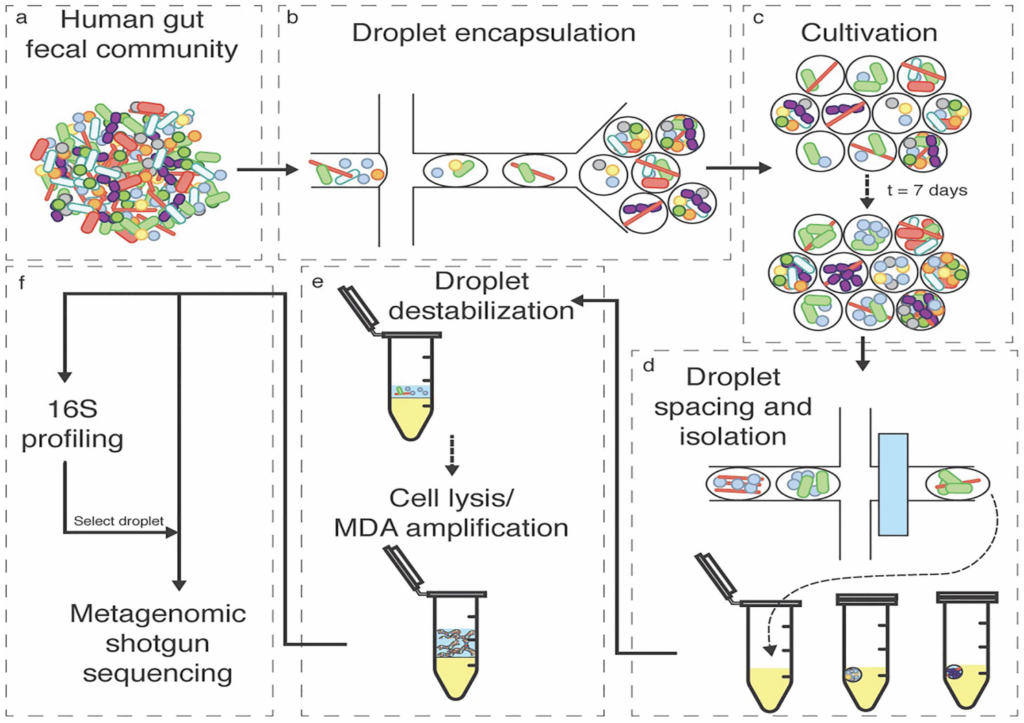
Our lab has been developing and applying new approaches and tools for the investigation of naturally occurring microbial consortia in order to discover the design principles underlying these complex systems. In particular, we have pioneered a technological pipeline, based on nanoliter-scale microfluidic droplets, to co-cultivate sub-communities and characterize member interactions that shape the structure and function of the microbial consortia. Several technological modules have been created and the pipeline is being applied to the investigation of a range of health or environment related microbiomes (see figure).
Professor Becky Lindsey
Our group combines machine learning and simulation to determine how materials behave at the atomistic scale, and exploit resulting insights for design, discovery, and optimization of materials for a broad range of applications. Student working in our group will have the opportunity learn to conduct simulations, use machine learning tools, and/or write code. For more information on our group, visit the Lindsey Lab website.
Project 1: Simulation-Guided Optimization of Membranes for sustainable ammonia synthesis
Sustainable fertilizer production is critical for feeding the earth’s ever-growing population. However, current processes used for industrial production of ammonia (a key component in fertilizer) is far from energy efficient. To help overcome this grand challenge, we use simulations to help guide design of the “next generation” of nanoporous membrane materials used as, e.g., catalytic supports for ammonia synthesis and “filters” for separating ammonia from reactants and by products.
Project 2: Machine Learning for “quantum accurate” simulation on large scales
Atomistic simulations rely on models that describe how system energy changes as the atoms within it move around. Historically, these models have either been based on quantum mechanical methods (which allow very accurate simulation of 100s of atoms), or molecular mechanical models (which allow relatively low accuracy simulation of millions of atoms), limiting utility of atomistic simulations. To overcome this challenge, we develop machine learned models that allow us to simulate with “quantum accuracy” on the large time and length scales accessible to molecular mechanics approaches. Ultimately, these advances allow us to study complicated chemistry-driven processes on scales directly overlapping with what experiments can probe, and let us watch how these systems evolve atom-by-atom. Students working on this project will have the opportunity to learn how to generate these models, and to contribute to advancements in our machine learning framework.
Project 3: Understanding nanocarbon synthesis under extreme conditions
Suddenly subjecting a carbon rich material “precursor” to extreme temperatures and pressures can drive formation of interesting and technologically relevant nanomaterials. In fact, “detonation synthesis” (whereby the material precursor driven to explode) is industrial process by which nanodiamonds are formed, when large quantities are needed. However, this process is dangerous, messy, and far from tunable. Instead, our group uses computer simulations to learn how we can apply similar principles to synthesize new and exotic nanocarbon particulates in a precise way (e.g., using lasers that allow us to drive materials to the relevant temperature and pressures in a finely controlled way) and to understand the fundamental chemistry and physics driving this process.
Professor Albert Liu
Colloidal Robotics: Functional self-assembly of distributed devices into integrated electrical networks
Functional self-assembly is the autonomous organization of components into task-oriented systems. The ability to overcome the entropic barrier to form hierarchical structures is an essential process in biology as well as the overarching objective of colloidal robotics. The goal is to develop higher order organizations of colloidal robotic devices with spatial heterogeneity, exhibiting ensemble-level functional utilities that push the limit of traditionally inanimate systems at the microscale. Specifically, we will leverage recent advances in both top-down (e.g., additive 3D printing) and bottom-up (e.g., guided self-assembly) techniques for colloidal robotic device integration in a distributed manner.
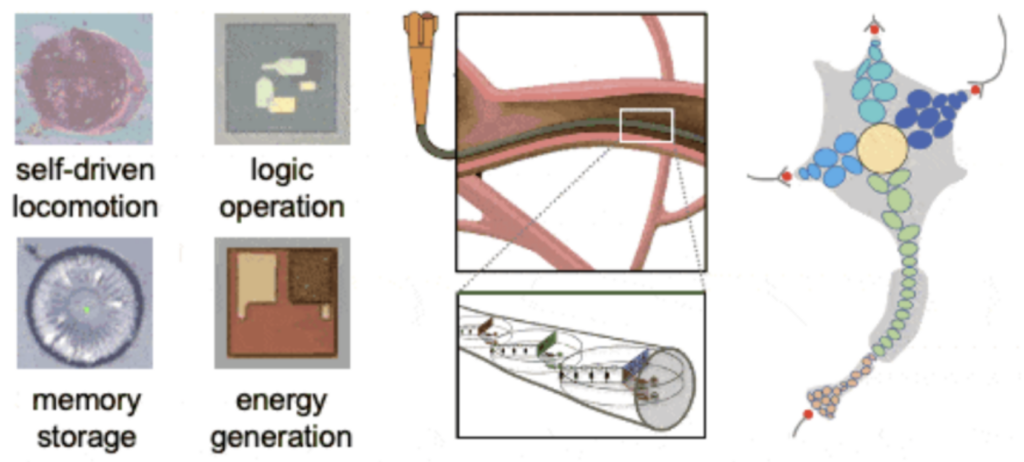
Most functional materials today are single-level assemblies of monomeric units. In contrast, biological systems have taken a multi-layered approach (i.e., organelles → cells → tissues, etc.), allowing multiple control checkpoints to be established at different levels. Our on-going efforts include incorporating colloidal robotic devices (which themselves consist of modular components with specialized functions) within existing materials such as fabrics and paint. Colloidal ink mixtures composed of minuscule electronic devices can be extruded into fibers, and subsequently weaved into textiles. When dispersed in solution or a polymer matrix, these electronic colloids can also be directly sprayed onto various surfaces.
Biology is replete with examples of dynamically assembled structures such as flocks of birds or foraging ant colonies. Translating these versatile organizations into inanimate systems requires an understanding of how dissipation of energy can lead to the emergence of ordered structures from disordered components. Because the components in dynamic self-assembling systems interact with one another nonlinearly, their behavior is often complex. As an early example, we fabricated a system of chemo-mechanical relaxation oscillators that exhibit an emergent self-synchronized rhythmic beating at the micro-scale. This complex system-level oscillation emerging from particles sharing a deceptively simple physical design exhibits highly tunable frequency. This simple chemo-mechanical oscillator provides a widely accessible experimental design to probe how rhythmic beating could come about amongst early multi-cell organisms, and illustrate how primitive entities could self-synchronize and exhibit complex collective behaviors.
For more information, visit the LEGOS Lab website.
Professor Sunitha Nagrath
Developing Microfluidic Systems for the Isolation of Circulating Cell Populations From Blood
Liquid biopsies or the process of using fluid samples, such as blood, collected from patients for the detection and study of a disease offers unique benefits for improving patient treatment. Blood draws are already a common procedure in clinical settings and are less invasive than other biopsy methods such as tissue biopsy. To study specific diseases, it is necessary to isolate disease specific biomarkers from the blood. This can include isolating diseased cell for both the detection and characterization of the disease. Isolation of these populations can be done using specially designed microfluidic devices that take advantage of specific cell characteristics including size or surface marker expression. The project will involve the design, fabrication, and/or experimental testing of microfluidic devices for cell isolation. Experimental testing will include the optimization of multiple device parameters and quantifying the device performance using specificity and sensitivity measurements. The devices in this project may be in the early stage of development or later stages of devices testing and optimization.
Isolation and Characterization of Circulating Tumor Cells from the Peripheral Blood of Cancer Patients
Metastasis is a complex, multi-step process which includes tumor cells enter the circulatory system, move throughout the body in the blood, enter into surrounding tissue, and finally proliferate to form secondary tumors. As metastasis form, they cause an increased health burden to patients and require the patients to undergo additional treatment and monitoring. It is estimated that metastasis are responsible for 90% of cancer deaths. To develop a deeper understanding of this process and to provide clinicians with patient specific information that may affect the patient’s course of treatment, it is necessary to do an in depth investigation of these circulating tumor cells (CTCs) from cancer patients. The project will involve the isolation and analysis of CTCs using various technologies. Blood collected from cancer patients will be processed on microfluidic devices, such as the Labyrinth and graphene oxide chip, to isolate CTCs. Once isolated, CTCs will undergo enumeration and molecular profiling to determine specific characteristics of the cells. These analyses will provide important insight into the heterogeneity of CTC populations, the process of metastasis, and provide clinicians with information about how the cancer is progressing so they can provide patients with the best treatment option.
Microfluidic Systems for Extracellular Vesicle Isolation – Microenvironment Influence and Metastasis
Metastasis is no longer viewed as a linear cascade of events but rather as a series of concurrent, partially overlapping processes, as successfully metastasizing cancerous cells assume new phenotypes while jettisoning older behaviors. The lack of a systemic understanding of this complex phenomenon has limited progress in developing treatments for metastatic disease. Although, rediscovery of extracellular vesicle and their many roles in the metastatic process, has further complicated the metastatic microenvironment, it has also provided some insightfulness towards phenomenon. Extracellular vesicles (EVs), a class of heterogeneous membrane vesicles, are generally divided into exosomes and microvesicles on basis of their origination from the plasma membrane. EVs facilitate the bidirectional communication among various cell types that can aid in various cell-to-cell and cell-to-microenvironment interactions. Specifically, tumor-secreted EVs (TEVs) are critical mediators of intercellular communication between tumor and stromal cells in local and distant microenvironments. Accordingly, TEVs play an essential role in both primary tumor growth and metastatic evolution. TEVs orchestrate multiple systemic pathophysiological processes, such as coagulation, vascular leakiness, and reprogramming of stromal recipient cells to support pre-metastatic niche formation and subsequent metastasis. The research project is to develop a microfluidic platform for the specific isolation and characterization of TEVs, to study how these vesicles facilitate metastatic process. Additionally, TEV bioactive constituents have been shown to have immunostimulatory/immunomodulatory properties. This requires detailed analysis of the influence that these TEV have on immune cells (e.g., T-cell and NK cells).
Investigation of Extracellular Vesicles in Blood Samples of Cancer Patients
Metastasis can be described as a multi-step phenomenon involving the release of cells from the primary tumor and their diffusion through the body. Currently, several hypotheses have been put forward in order to explain the origin of cancer metastasis, including epithelial–mesenchymal transition, mutagenesis of stem cells, and a facilitating role of macrophages, involving, for example, transformation or fusion hybridization with neoplastic cells. In this paradigm, TEVs play a pivotal role in cell communications, delivering a plethora of biomolecules including proteins, lipids, and nucleic acids. For their natural role in shuttling molecules, EVs have been considered an integral part of the metastatic cascade. They have a prominent role in preparing the tumor microenvironment (TME) in target organs. However, we require a more detailed understanding of the packaging of TEV constituents and on the constituents themselves. To develop a deeper understanding and framework for the therapeutic capabilities of TEVs, it is imperative to do an in-depth investigation of TEVs from cancer patient. This investigation will include isolation of patient TEVs and identifying the various subgroups of TEVs expressed in their blood. Upon isolation of TEVs subgroups, investigating their immunostimulatory/immunomodulatory capabilities a therapeutic option will follow. This project involves the evaluation of TEVs as a patient-specific immunotherapeutic option for pre-metastatic cancer patients. In this project, we will study the interaction of TEVs and T-cells to evaluate the uptake and transfer of bioactive constituents. We plan to measure any immune activation response of the T-cell by measuring the expression levels of known immunostimulatory molecules (ex. IL-2 and IL-10). These analyses will provide important insight into the goal of TEVs as a personalized treatment option for pre-metastatic patients.
Professor Nirala Singh
Electrocatalytic bio-oil hydrogenation
Bio-oil hydrogenation is a method to produce carbon-neutral jet fuels if catalysts can be developed that are active and stable. This work will consist of studying electrocatalysts for these hydrogenations and documenting their performance.
Professor Michael Solomon
Synthesis and Assembly of Nanocolloids
Nanocolloids are constituents of materials that are applied in many areas such as inks, coatings, optical materials, sensors and drug delivery. These particles are smaller than about one micron. In this project you will learn methods to synthesize, assemble and characterize such particles. The synthesis procedures you will master include methods to produce monodisperse particles. You will learn how to characterize what you make by electron and confocal microscopy. You will assemble particles by means of sedimentation, spin coating or applied electric fields. You will gain experience characterizing the rheological and electrokinetic properties of the particles you synthesize. At the conclusion of the project you will be well prepared to work or perform research in the many areas and industries that work with colloidal particles.
Physical Characterization of Bacterial Biofilms
To survive in the many environments they inhabit, bacteria may grow in communities in which individual organisms are embedded in a polysaccharide matrix. Biofilms are examples of such communities. Biofilms adhere to a variety of surfaces, including substrates relevant to human health, such as catheters. Confocal microscopy is a tool widely used in microbiological research because it can resolve multiple fluorescence emissions in three dimensions. Our aim here is for students to apply microscopy methods to characterize the microstructure of bacterial biofilms and aggregates. Students participating in the project will receive training in microscopy, computer image processing, and bacterial communities. This unique combination of research in soft matter/complex fluids and microbiology will provide students with a strong foundation from which to pursue subsequent research experiences and graduate training.
Professor Peter Tessier
Bioinformatics and computational methods for improving antibody discovery
The success of therapeutic antibodies depends not only on their specific bioactivities but also on their highly variable and difficult-to-predict physicochemical properties (solubility, specificity, and biodistribution). The goals of this project are to develop predictive computational and bioinformatics methods for designing, optimizing and identifying drug-like monoclonal antibodies for therapeutic and diagnostic applications. Interested candidates should have experience in programming and MATLAB, and an interest in biotechnology.
Professor Greg Thurber
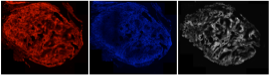
The human body can be treated as a large Chemical Engineering transport problem, where oxygen, nutrients, and drugs distribute based on a multitude of factors including passive transport (e.g. diffusion) and active mechanisms (e.g. drug transporters or ‘pumps’). By utilizing simulations of drug transport across multiple length and time scales, novel diagnostic imaging agents and therapeutics can be ‘designed’ rather than ‘screened,’ improving the efficiency in development.
Two areas in particular remain poorly understood: transient distribution of small molecule imaging agents and therapeutic distribution of novel biologics. Our lab is interested in integrating drug and physiological factors into simulations for designing more effective imaging agents and therapeutics. Transport across multiple length and time scales must be included in detailed simulations to predict the impact of drug delivery mechanisms and therapeutic design, from the whole body and organ level down to the tissue, cellular, and subcellular distribution of the drugs.
Novel Breast Cancer Imaging Agents for Early Diagnosis
Our lab is developing novel orally available imaging agents for the early diagnosis of breast cancer – in other words, a disease screening pill. The critical parameters for designing these new agents include the oral absorption and the target to background ratio (TBR). A high TBR agent can detect smaller cancer lesions against the background of normal tissue, allowing a surgeon to intervene early, resulting in better outcomes. The TBR is a complex function of passive diffusion through tissue, active binding to the target of interest, and clearance by the kidneys and other organs. In this project, the student will run computational simulations of imaging agent distribution as a function of molecular properties. If in-person work is possible, they will measure the specific binding affinity of novel imaging agents against a series of cancer cells grown in the lab and measure passive transport rates across cell barriers (e.g. endothelial cells lining blood vessels). These rates can then be fed back into the simulation to predict the optimal properties.
Professor Angela Violi
The VioliGroup is a computational chemistry and physics group that specializes in simulations and machine learning for nanoparticle formation, protein-nanoparticle interaction, nanoparticle transport across bacterial membranes, and biofilm characterization.
Designing Nanomaterials for Energy and Biological Applications
Our lab is currently looking to hire a student to help develop and run code for computational (nano)chemistry. Some experience with computational chemistry, Python programming and Linux Terminal is important. In our lab, such a student would gain experience with molecular dynamics simulations, geometric deep learning, stochastic molecular simulations, combustion simulations, nanoscale interaction prediction, and generative nanoscale/molecular design.
If you are interested, please contact Prof. Violi.
Professor Robert Ziff
The Study of Networks and Percolation
Many problems in science and engineering involve random networks and porous media. When the connection is sufficiently high, long-range flow is allowed and the system is known to “percolate.” Related problems include electrical conductivity of random conductor/insulator mixtures, dissolution of pills that are made of soluble/insoluble aggregates, and the spread of disease though a population of susceptible and unsusceptible individuals.
These various problems can be modeled by a simple and elegant probabilistic geometrical model in which a regular lattice is made random by making some of the edges or “bonds” open or shut.
The project is to study various forms of these networks through computer simulation and mathematical analysis, to determine connectivity and transport processes, especially near the critical threshold. If you are interested in programming, the project can entail running jobs on a computer (based upon current programs, modified accordingly), and analyzing the results.
Other aspects of this project include literature searches, assistance in manuscript preparation (especially figures), and additions to web and Wikipedia pages on this subject. Several previous undergraduate student projects have resulted in publications in journals.